Photon-tagged jet quenching in the quark-gluon plasma
23 October 2017 | By
Collisions of lead nuclei in the LHC form the hot, dense medium known as the quark-gluon plasma (QGP). Experimentally, the QGP is characterised by the collective flow of emerging quarks and gluons. They fragment into highly collimated “jets” of particles that in turn lose energy through a phenomenon known as “jet quenching”. Studying this effect can help improve our understanding of quantum chromodynamics, the theory of the strong nuclear interaction that governs the behaviour of the QGP.
In ATLAS, jet quenching has been measured using a variety of techniques. In one method, the total production rates of large transverse momentum jets are found to be substantially suppressed in “central” lead-lead collisions (those in which the colliding lead nuclei have a large overlap and create an extended region of QGP). Jet quenching has also been observed in individual events, such as when the expected momentum balance between pairs of jets is found to be distorted by the presence of the QGP medium.
Events in which a jet is produced opposite a high momentum photon are particularly useful since the photon does not appreciably interact with the quarks and gluons composing the medium (see Figure 1). The fraction of the photon’s momentum carried by the balancing jet in lead-lead collisions has been measured by ATLAS, and was found to be strongly shifted to lower values, reflecting the attenuation of the jet’s total momentum as it passes through the medium.
In addition to quenching the overall jet momentum, the medium can also distort how the remaining momentum is spread among the hadrons in the jet. These so called “fragmentation functions” have been measured for the first time by ATLAS for jets opposite to a photon in proton-proton and lead-lead collisions.
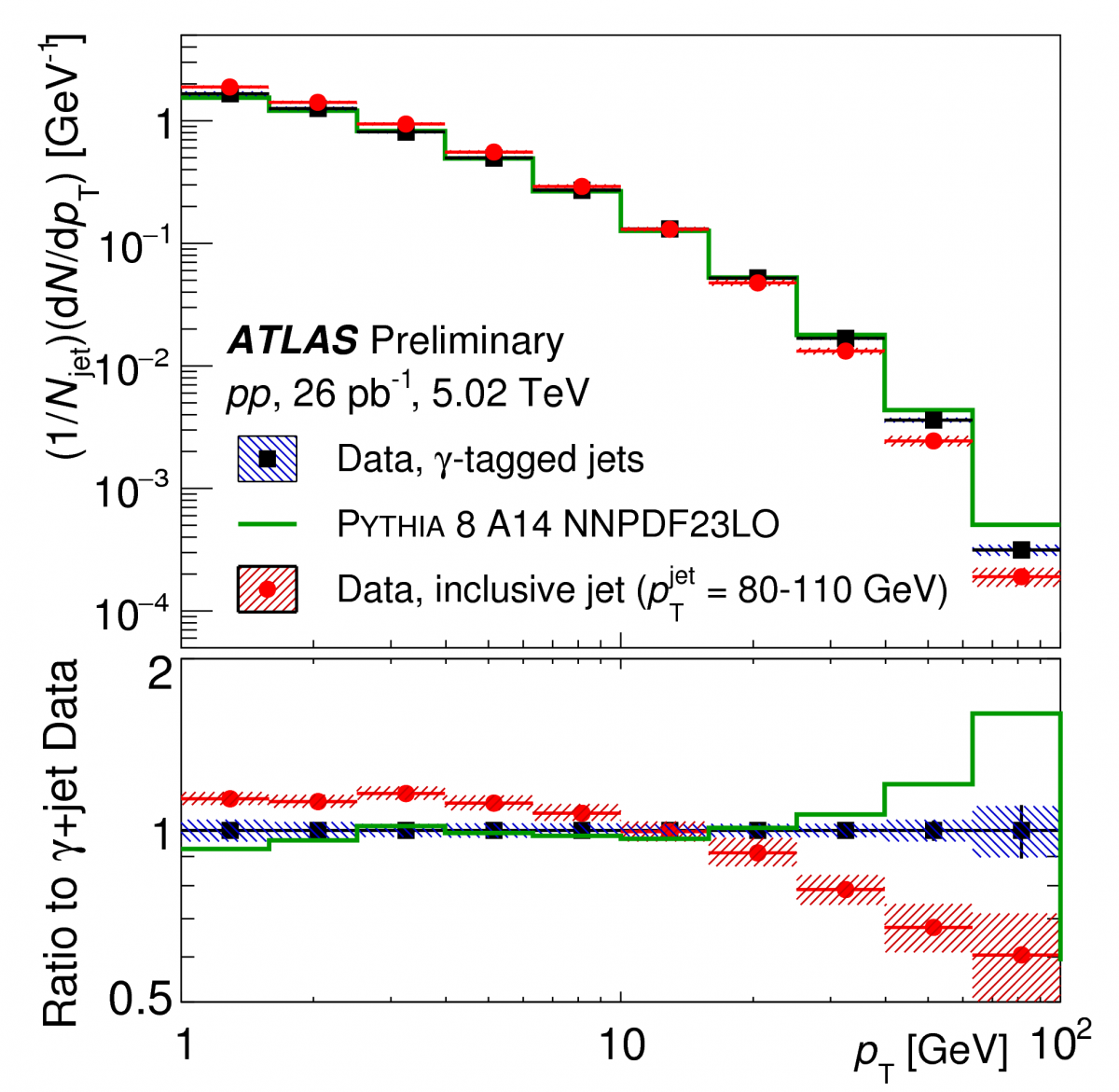
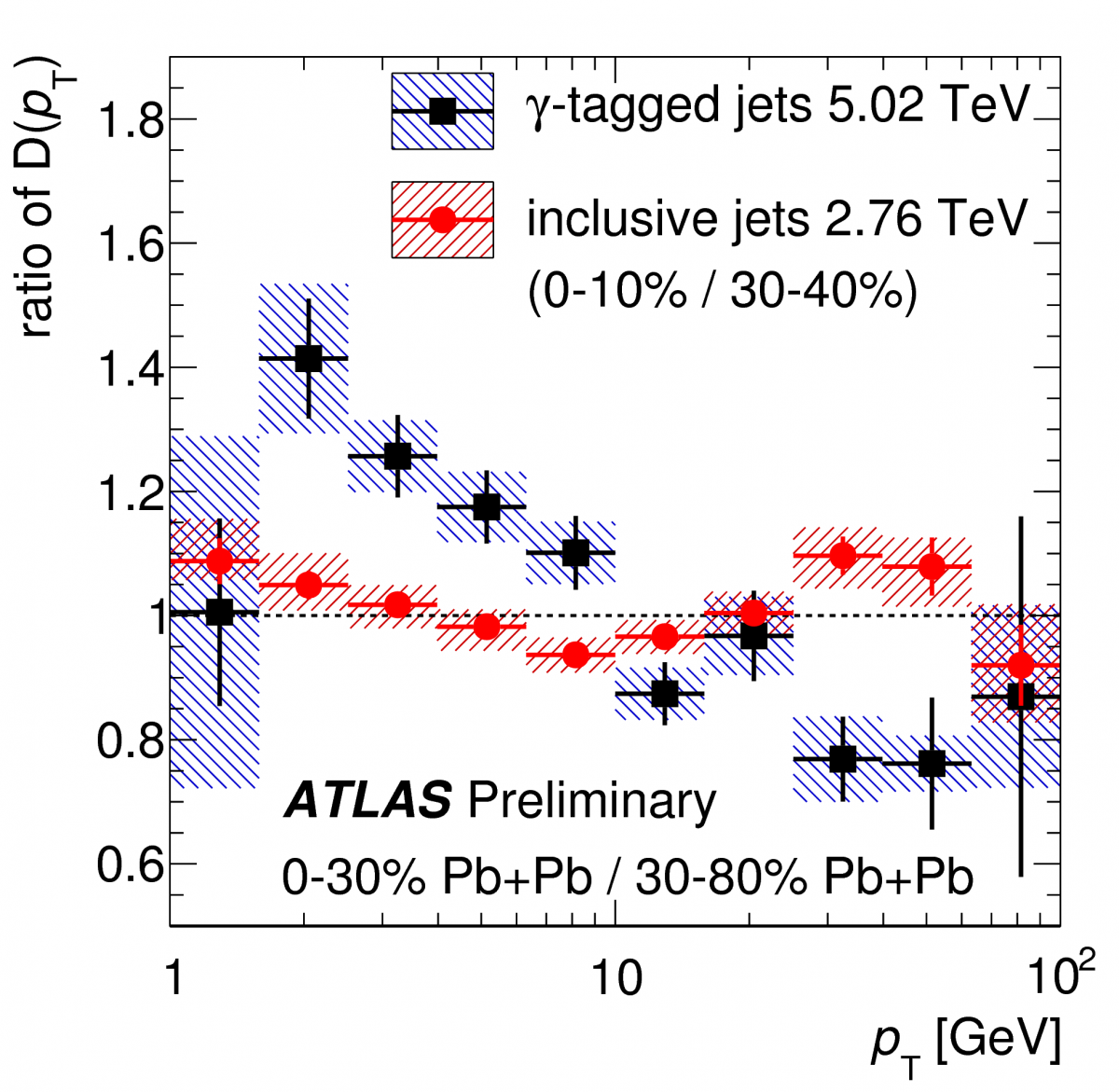
Photon+jet events are most likely to arise from the Compton scattering of a gluon in one of the beams off a quark in the other beam, meaning that the jet opposite the photon was most likely initiated by a fragmenting quark. By contrast, jets with a similar momentum but without an associated photon are more likely to come from fragmenting gluons. Figure 2 compares these different fragmentation functions in proton–proton collisions, shown here as a function of the transverse momentum of the hadrons inside a jet. Jets initiated primarily by a quark have a fragmentation pattern that is more likely to create more energetic fragments than that of the gluon-majority jets, as expected from previous studies of quark and gluon jets.
In peripheral lead–lead collisions (where the nuclei have modest overlap and create a moderate-sized QGP region), the fragmentation function for photon-balancing jets is found to be significantly modified compared to that in proton–proton collisions, reflecting the effects of the distorting medium. In central lead–lead collision events, these modifications are found to be even larger. This is shown in Figure 3, which compares the ratio of the fragmentation function in central to peripheral lead–lead events, for both types of jets. These results suggest that as jets travel through a larger and hotter QGP region, their internal structure is systematically further modified.
Intriguingly, studies of the fragmentation function for inclusive jets observe a different behaviour – which is that past a certain QGP size, the emerging jets do not continue to be modified. Since this unexpected feature of the data may arise from a number of factors, more detailed studies with the higher photon+jet statistics expected in the 2018 lead–lead data-taking will be useful to reveal the origin of this effect.
Links:
- Measurement of the fragmentation function for photon-tagged jets in 5.02 TeV Pb+Pb and pp collisions with the ATLAS detector (ATLAS-CONF-2017-074).
- Measurements of the Nuclear Modification Factor for Jets in Pb+Pb Collisions at 2.76 TeV with the ATLAS detector (Phys. Rev. Lett. 114 (2015) 072302, see figures).
- Measurement of jet pT correlations in Pb+Pb and pp collisions at 2.76 TeV with the ATLAS detector (arXiv: 1706.09363, see figures).
- Study of photon-jet momentum correlations in Pb+Pb and pp collisions at 5.02 TeV with ATLAS (ATLAS-CONF-2016-110).
- See also the full lists of ATLAS Conference Notes and ATLAS Physics Papers.