Technology Transfer
Many cutting-edge technologies developed for the ATLAS Experiment have found new applications in other fields
Studying vision
A team of ATLAS collaborators developed a system that can record the neural activity of retinal cells.
Inspired by the silicon microstrip detector technology employed in ATLAS, the team created an advanced multi-electrode array system that can simultaneously record the neural activity of hundreds of the retinal output (ganglion) cells.
The heart of the system is a two-dimensional array of 512 microscopic electrodes densely packed together in an area of 1.7 mm2 (about the size of a pinhead). Over the years, additional designs have been implemented, allowing neurobiologists to study retinal cells with a variety of spatial densities.
This system led to the discovery a new functional type of retinal ganglion cell. They are believed to play a role in the perception of motion. These cells are rare and difficult to spot with traditional one-electrode technology.
Researchers are now applying this ATLAS technology to retinal prosthesis studies, to convey visual information to the brain when photoreceptors are degenerated. But that’s not all – similar technology is now being used to study the electrical activity of neurons in the brain, helping to reveal how visual information is processed and encoded.
HADRON THERAPY
Diamond sensors used in the ATLAS detector have been successfully applied to hadron therapy.
Diamond sensors are made of synthetic diamonds, called CVD (chemical vapours deposition). They are installed in ATLAS’ Beam Conditions Monitor and Diamond Beam Monitor, in the pixel detector region. They have many advantages for their application as beam monitors: increased resistance to radiation, a quicker response time and the ability to operate at room temperature. These qualities make them ideals as tools for hadron therapy. Hadron therapy is a medical treatment that uses charged particles (like protons or carbon ions) to irradiate tumours, killing the cancer cells while trying to spare the surrounding healthy tissues. Diamond sensors are used to precisely monitor the particle beams in hadron therapy, measuring their intensity and time structure.
ULTRASOUND GAS ANALYSIS
Ultrasonic instruments, developed to monitor the composition of gas mixtures in the ATLAS silicon tracker cooling environment, are currently being adapted as tools for clinical anaesthesia.
These devices transmit ultrasonic pulses through a mixture of two gases, measuring their propagation times in opposite directions. Since sound velocity is affected by the composition of the medium, the instrument is then able to estimate the ratio of the gases in the mixture and can simultaneously measure the gas flow rate.
This technology was developed to detect the presence of leaks in the ATLAS inner detector cooling system. This system uses two coolant fluids: octa-fluoropropane (C3F8) for the SCT and Pixel sub-detectors and carbon dioxide (CO2) for the Insertable B-Layer. Surrounding these sub-detectors are volumes of nitrogen that help to minimise the presence of water vapour in the detector.
Using these ultrasonic devices, ATLAS engineers can detect the presence of C3F8 and CO2 in the nitrogen volumes, revealing if there are any leaks in the system.
Researchers are now exploring possible biomedical applications. In particular, the device could be used to control the composition of anaesthetic gases in real time. It is especially suitable for a new type of anaesthesia that uses mixtures of xenon and oxygen, which normally require two different measurement techniques to analyse.
MEDICAL IMAGING
3D silicon sensors developed for the ATLAS Insertable B-Layer could have a bright future in medical imaging.
Unlike traditional sensors, the electrodes in 3D sensors are processed inside the silicon bulk instead of being implanted on the wafer’s surface. This particular structure gives them an enhanced resistance to radiation, and for this reason they were developed specifically for the ATLAS pixel region.
If used in medical imaging, one of the most important characteristics of 3D sensors would be their ability to provide a high image resolution with low-dose radiation. This could be beneficial as exposure to X-rays is potentially dangerous and, consequently, the radiation dose should be as low as possible. These sensors could also deliver fast and focussed signals - useful for X-ray imaging and Positron Emission Tomography (PET) - and precise volume definition - needed in micro-dosimetry during cancer therapy.
Scientists are experimenting with other possible applications, including neutron imaging, a scanning technique that uses beams of neutrons instead of X-rays.
SOUND REPRODUCTION
Inspired by ATLAS’ optical metrology technology, a team of researchers developed a new system to recover sound from old records.
In ATLAS’ SemiConductor Tracker there are 16,000 carefully aligned silicon detectors. Their accurate position was obtained thanks to optical metrology tools, which can give measurements with a precision of a few micrometres.
This same technology was applied to audio digitizing by a team of scientists from Lawrence Berkeley National Lab, headed by ATLAS collaborator Carl Haber. They developed a machine (called IRENE) which is able to extract sounds from old audio devices such as phonograph discs and cylinder records. These devices represent sounds as grooves on their surface. Optical metrology was used to reconstruct the exact shape of the grooves and, consequently, to create a digital version of the recording. All this was achieved without actually touching the records, so that there is no risk of damage.
Haber’s team restored many important historical recordings. For instance, thanks to IRENE we were able to hear Alexander Graham Bell’s voice for the first time.
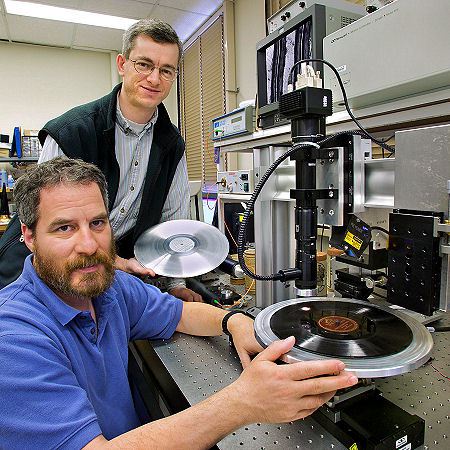