ATLAS highlights presented at the world's largest particle-physics conference
8 August 2020 | By
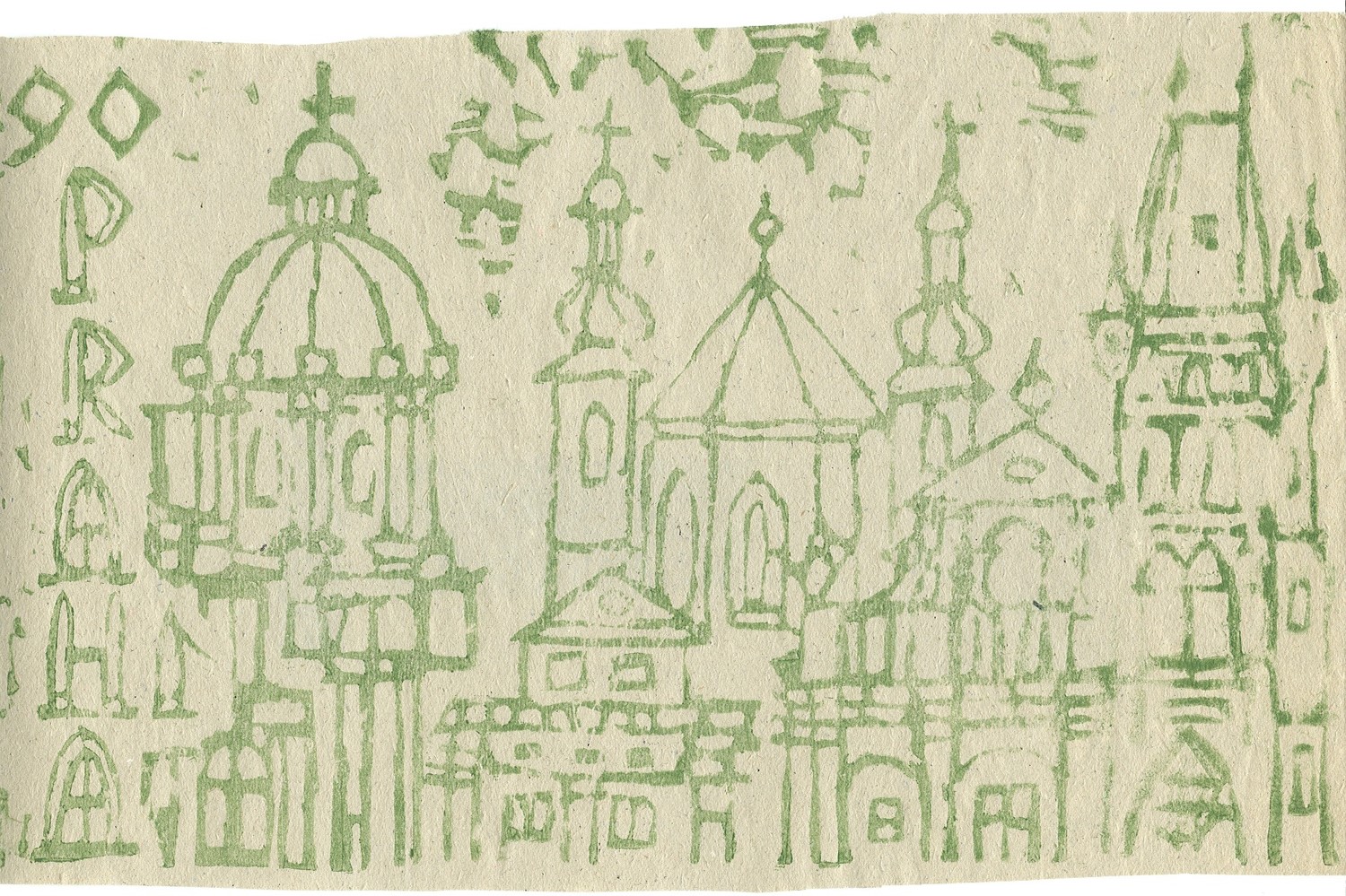
Instead of gathering in the beautiful city of Prague, particle physicists had to meet online for the 40th International Conference of High Energy Physics (ICHEP 2020). ICHEP, the largest conference in particle physics, takes place biannually and brings together delegates from all fields of particle and astroparticle physics. Traditionally, the first three days are dedicated to parallel sessions presenting results in detail, with the remainder of the conference focusing on summary plenary talks by experts. To better accommodate attendees from the different time zones, the conference was slightly prolonged with parallel sessions from Tuesday 28th to Friday 31st July and plenary sessions from Monday 3rd to Thursday 6th August. The sessions took place each day either during the European morning or late afternoon, with replay options available. Also virtual poster sessions, where young physicists could present their work, were held.
As major players in the field of particle physics, the LHC collaborations contributed many new results, most of which exploited the full Run 2 dataset, recorded in 2015 to 2018. ATLAS physicists contributed 35 new results, and gave 85 talks in the parallel and plenary sessions. Their contributions spanned a wide range of topics, from precision measurements and searches for new phenomena to detector performance and R&D, as well as diversity and outreach.
From precision measurements to searches for new phenomena, the ATLAS Collaboration presented a wealth of exciting new results at ICHEP 2020.
Two-photon production
Two highlights this year concern photon production in proton–proton collisions. In “normal” collisions at the LHC, a quark or a gluon from one proton interacts with a quark or a gluon from a proton in the opposing beam, breaking the two protons apart. However, in the rare cases where the protons pass close by each other instead of colliding head-on, each proton can radiate a photon which can then collide while the proton stays intact. This results in events where, except for the particles stemming from the photon–photon interaction, the ATLAS detector sees no other particles originating from the primary interaction vertex.
By studying these unique events, ATLAS physicists have for the first time observed the production of two W bosons from the collision of two photons. This process can only happen via the interaction of three or four gauge bosons. ATLAS physicists observed this process in events where one W boson decays into an electron and an invisible neutrino, and the other into a muon and a neutrino. Figure 1 shows the number of additional charged-particle tracks pointing to the electron–muon interaction vertex, where one can see a clear enhancement at zero from the two-photon interaction events.
Photon-photon interactions have also been studied, for the first time, using ATLAS’ forward detectors. When a proton loses energy due to the photon radiation, it may be deflected by the LHC magnets into the new ATLAS Forward Proton spectrometer (AFP), situated 220 metres downstream along the beamline on either side of the experiment. The energy loss of the protons can be calculated from their position measured in the AFP.
When the photons decay into a pair of leptons (electrons or muons), the energy losses to the two protons can be calculated from the lepton kinematics and be compared to the AFP measurement. The observation of this effect was presented by ATLAS physicists at ICHEP. Figure 2 shows the difference of these two measurements for events with a clean lepton pair, where a proton was detected in one of the two AFP sub-detectors. Clear peaks at zero are visible, opening possibilities of additional interesting results in two-photon production during Run 3.
Higgs boson
For the ICHEP conference, ATLAS also released a plethora of new results measuring the properties of the Higgs boson. Up until now, the Higgs boson had only been seen coupling (interacting) with gauge bosons and third-generation fermions. Yet the Standard Model predicts that the Higgs boson couples to all fermions with a strength proportional to their mass. A new ATLAS paper presents the search for Higgs boson decays into two muons, of the second fermion generation. This decay is not only extremely rare but there is also a huge background from other Standard Model processes that also create muon pairs. Figure 3 shows the di-muon mass spectrum around the Higgs-boson mass. A small excess of 2 standard deviations above the no-Higgs hypothesis can be seen, giving a first indication that the Higgs boson couples indeed also to the second generation of fermions.
In just the first half of this year, the ATLAS Collaboration had already published several Higgs-boson analyses using the full Run 2 dataset. For ICHEP, the collaboration added a detailed measurement of Higgs boson properties in the two-photon decay channel, and the observation of vector-boson-fusion production in the W-boson pair decay channel. This almost completes the set of major production and decay channels with the full dataset. The available data were combined and interpreted under various assumptions, and in all cases are in good agreement with the Standard Model.
The combination also established the observation of associated production of a W boson and Higgs boson, the only major Higgs-boson production process missing until now. Assuming that the Higgs boson decays only into known particles, the coupling constants to weak bosons, photons and gluons could be measured with a precision of better than 10% and, in some cases, the measurements are as precise as the theoretical prediction. For example, Figure 4 shows the coupling strength of the Higgs boson couples to Standard Model particles. It clearly shows that the Higgs field fulfills its role of generating mass as predicted in the Standard Model.
Another new study constrained the fraction of Higgs-boson decays into invisible particles or into decay channels that cannot be measured by the detector. By carrying out a direct search for invisible Higgs-boson decays using events with two forward jets and missing transverse momentum, ATLAS physicists limited the fraction of invisible Higgs-boson decays to 13% (at 95% confidence level). Then, by combining all Higgs data, this was further improved to 9%.
Standard Model measurements
The ATLAS Collaboration also presented several precise measurements of Standard Model processes. With the large Run 2 dataset it is even possible to measure rare processes in detail, while processes with higher cross sections can be split into many different regions. For top-antitop events where an additional Z boson is radiated, physicists measured differential distributions such as the transverse momentum of the Z boson. This process is difficult to calculate theoretically, but a reasonable agreement between the measurement and theoretical predictions was found.
Physicists also studied the production of four leptons, a process with very rich phenomenology. Depending on the invariant mass of the four leptons, the dominant underlying process behind their creation can be the decay of a Z boson, a Higgs boson that decays via two Z bosons, or the electroweak production of a Z-boson pair. The ATLAS Collaboration measured the invariant-mass spectrum of four-lepton events and obtained differential distributions separated in mass regions each corresponding to a dominant production process. These distributions allowed physicists to set limits on new physics.
The large dataset collected during Run 2 of the LHC made it possible for ATLAS physicists to measure rare processes in detail.
Searches for new phenomena
Researchers also released important updates on the search for new phenomena. One of the greatest puzzles in physics today is the nature of dark matter. ATLAS presented new full Run-2 results for the “work-horse” analysis for dark matter searches: the monojet search. If dark-matter particles are produced at the LHC, they escape the experiments undetected. A hadronic jet may either be produced in the hard interaction or can come from initial-state radiation. So, in principle, each process where dark matter is produced should be visible in a search for a jet and missing transverse momentum. Figure 5 shows that the missing-transverse-momentum spectrum is well described by known processes and no sign for dark matter is seen. To transfer the absence of a signal into exclusion limits, physicists looked at concrete theoretical models. For example, if one assumes that dark-matter production is mediated by a new gauge boson with axial vector couplings and specific assumptions on the coupling strengths, a mass limit of up to 2 TeV can be set for this gauge boson.
Models that describe the unification of forces at high energy often require the existence of leptoquarks, heavy particles that carry the quantum numbers of leptons and quarks. These particles have recently regained popularity as they may explain some anomalies seen in studies of B physics. ATLAS physicists have searched for several types of leptoquarks (1, 2, 3) and set limits up to 1.8 TeV on the leptoquark mass.
A frequently studied extension of the Standard Model is supersymmetry. Supersymmetric models predict a scalar partner for every fermion, and a fermionic partner for every scalar or vector boson in the Standard Model. The formerly favoured variants of supersymmetry, where the partners of the gluons and light quarks are in the TeV range, have been excluded by LHC experiments. The large luminosity of Run 2 allowed researchers to attack more difficult scenarios where, for example, only the partners of the non-strongly-interacting particles are in the accessible energy range, or the mass difference between the (in principle) visible superpartner and the lightest supersymmetric particle is very small. No signal was found in these searches and stringent limits were set.
In the Standard Model, all lepton flavours couple identically to gauge bosons and lepton flavour is conserved to a very high degree. The ATLAS Collaboration tested both of these predictions with unprecedented precision. Using an unbiased W boson sample from top-quark decays, they measured the ratio of branching ratios of W bosons decaying into a muon and a neutrino or a tau and a neutrino. This was found to be compatible with unity with a precision of 1.3%. This result is a factor two better than the previous result which combined data from all of the LEP experiments and disagreed with unity at 2.7 standard deviations. In a second analysis, physicists searched for Z-boson decays into an electron and a tau or a muon and a tau. They found no evidence for such decays and limits of, respectively, 8.2 and 9.5 times 10-6 were obtained, overtaking the previous LEP limits by about 20%.
All of these new ATLAS results were well received in an overall successful conference. The organisers worked hard to make the online format as pleasant as possible but we look forward to meeting again in person - we will have another chance to see Prague during an ICHEP conference in 2024!
Links
- Summary of new ATLAS results for ICHEP 2020
- ATLAS observes W-boson pair production from light colliding with light, Physics Briefing, 5 August 2020
- New ATLAS result marks milestone in the test of Standard Model properties, Physics Briefing, 3 August 2020
- New measurements of the Higgs boson find strength in unity, Physics Briefing, 31 July 2020
- Looking forward: ATLAS measures proton scattering when light turns into matter, Physics Briefing, 30 July 2020
- ATLAS probes interactions between heavyweights of the Standard Model, Physics Briefing, 30 July 2020
- Jetting into the dark side: a precision search for dark matter, Physics Briefing, 28 July 2020
- ATLAS one step closer in the search for rare Higgs boson decays to muons, Physics Briefing, 23 July 2020
- New ATLAS result addresses long-standing tension in the Standard Model, Physics Briefing, 28 May 2020