The invisible plan
17 July 2017 | By
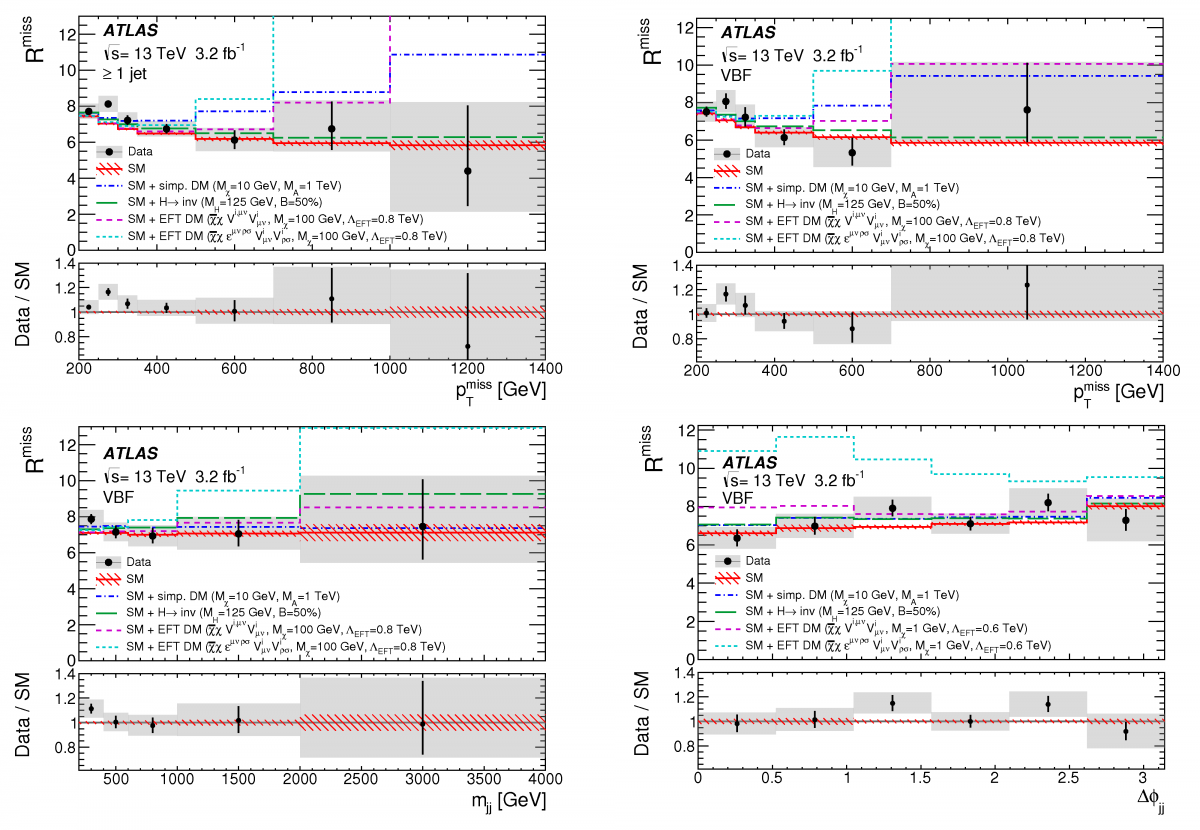
As the Large Hadron Collider (LHC) smashes together protons at a centre-of-mass energy of 13 TeV, it creates a rich assortment of particles that are identified through the signature of their interactions with the ATLAS detector. But what if there are particles being produced that travel through ATLAS without interacting? These “invisible particles” may provide the answers to some of the greatest mysteries in physics.
One example is Dark Matter, which appears to make up 85% of mass in the Universe but has not been conclusively identified yet. We learned of its existence through astrophysical observations, including galaxy formation and gravitational lensing. However, we know more about what it isn’t than what it is. There is no single theory of Dark Matter; different predictions have different implications for its properties and how it interacts.
The invisible particles produced in LHC collisions carry away energy, resulting in an apparent imbalance in the energy/momenta of the observed visible particles. Different theories predict that, if the invisible particles exist, more events with large imbalance and other distinctive patterns of visible particles could be detected by ATLAS. Comparing the number of such events predicted by theory to the number of events observed in the detector is a way of searching for invisible particles indirectly.
While shown to be a successful approach, there are limitations. What if all our theoretical models of Dark Matter are wrong? What if an entirely different phenomenon is the cause of invisible particles? Currently, if theoretical models are shown to be incorrect, it can be difficult and time-consuming to re-use the data to test new models. To do so requires an understanding of how these particles were recorded in the detectors, how the events were selected, and how the Standard Model processes that mimic these particle patterns were modelled.
ATLAS physicists have developed a new measurement-led approach, which is designed to be detector-independent and allows for easy re-interpretation of the data in future.
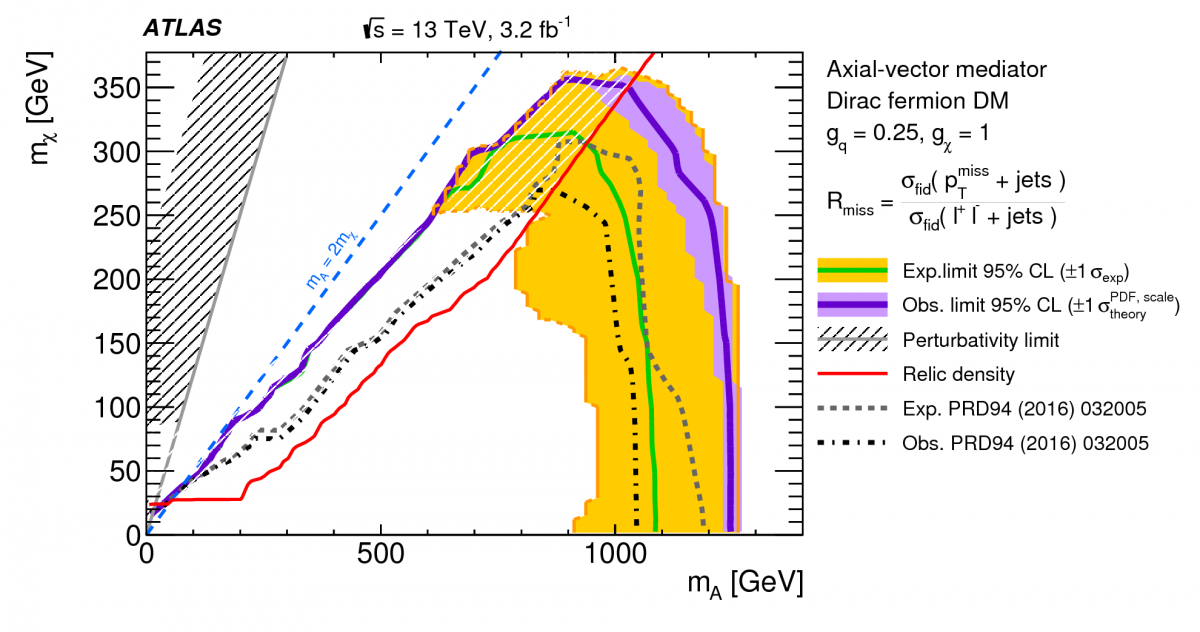
ATLAS physicists have developed a new measurement-led approach, which is designed to be detector-independent and allows for easy re-interpretation of the data in future. In this approach, a quantity Rmiss is defined which is sensitive to the production rate and properties of any invisible particle(s). This quantity is measured versus various properties of the collision events, including the amount of momentum imbalance and the energy/momenta of the visible particles. Not just the value of this quantity, but how it changes with these measured properties is found to provide sensitivity to invisible particles. Known decays of Z bosons – produced in LHC collisions – into invisible neutrinos mean this quantity is non-zero even in the absence of a new invisible phenomenon. This quantity is carefully corrected for detector inefficiencies, leaving a measurement free from experimental bias and independent of any new physics hypothesis (Figure 1). Any physicist can then easily compare the predictions of their model against this measurement.
To demonstrate the new approach, the measurement is used to test three distinctly different theoretical models of Dark Matter, where it is produced either (1) via the strong force, (2) through the decays of Higgs bosons, or (3) via the electroweak force. No evidence of Dark Matter is observed and so ATLAS is able to place stringent constraints on these theories (Figure 2). The constraints are competitive with existing approaches that aim to test these specific theories and complementary to measurements from space-based indirect detection experiments.
So, no matter what mysteries lie in wait in the “invisible” realm, ATLAS has the techniques it needs – both now and in the future – to continue to learn more about the Universe.
Links
- Measurement of detector-corrected observables sensitive to the anomalous production of events with jets and large missing transverse momentum in proton-proton collisions at 13 TeV using the ATLAS detector (arXiv: 1707.03263, see figures)
- See also the full lists of ATLAS Conference Notes and ATLAS Physics Papers.