How strange is the proton?
25 January 2017 | By
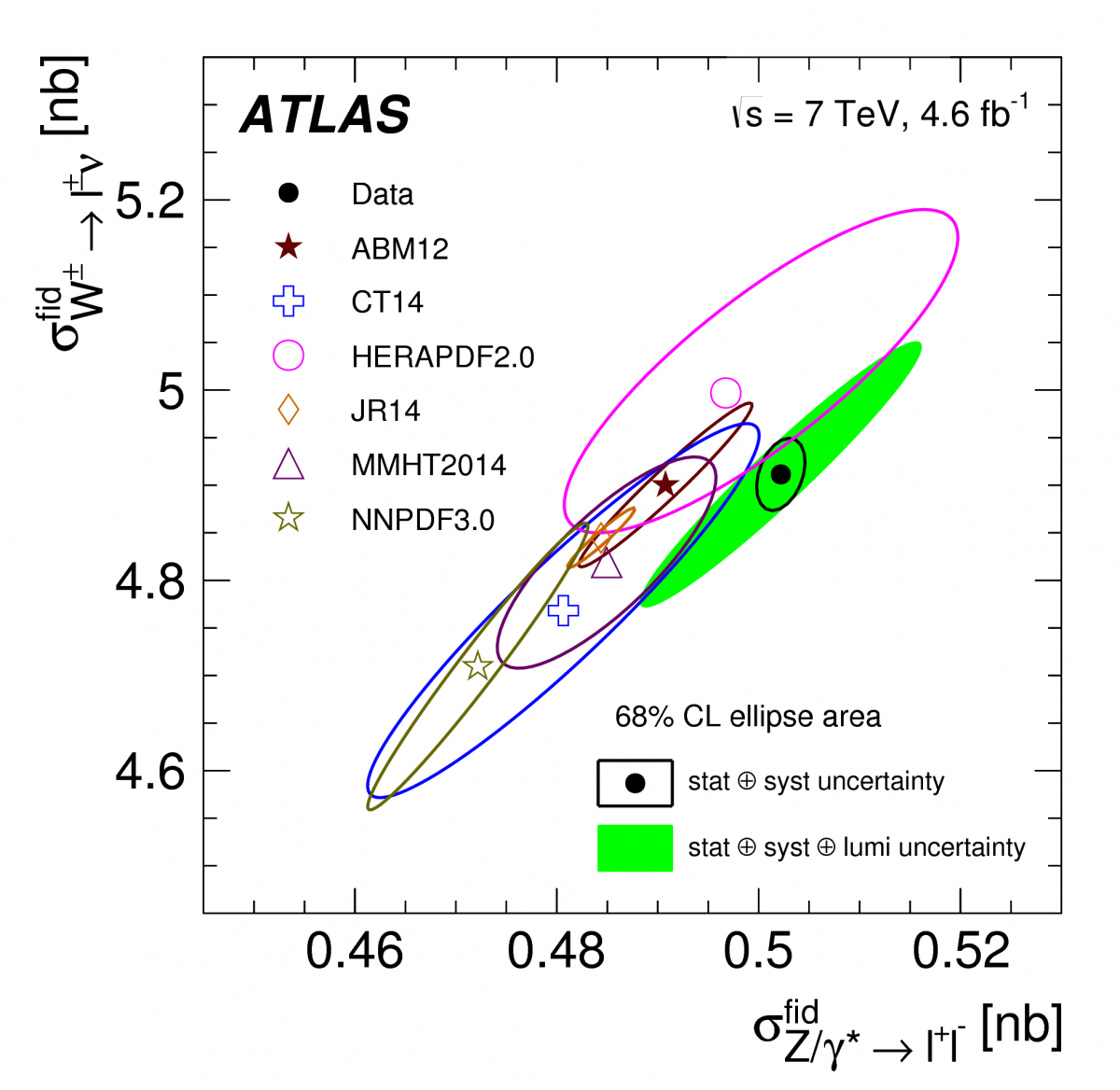
The protons collided by the LHC are not elementary particles, but are instead made up of quarks, antiquarks and gluons. The theory of the strong interactions – quantum chromodynamics (QCD) – does not allow physicists to calculate the composition of protons from first principles. However, QCD can connect measurements made in different processes and at different energy scales such that universal “parton density functions” (PDFs) can be extracted. These determine the dynamic substructure of the proton.
The discovery of quarks as the elements of the partonic structure of the proton dates about 50 years. Soon after QCD was born and the existence of gluons inside the proton was established. Much has since been learned through a combination of new experimental data and theoretical advances. At the LHC, reactions involve quarks or gluons that carry a certain fraction x of the proton’s momentum, expressed through the Bjorken x variable. Below x of 0.01, the proton constituents are mainly gluons and a sea of quark-antiquark pairs.
Electron-proton scattering data from the HERA collider has constrained the gluon and the sum of all quarks weighted by the square of their electric charge. But the low x sea-quark composition – expressed in terms of the lighter quarks named up, down and strange quarks – is still not well understood. New data from the ATLAS experiment shows, with unprecedented precision, the production of W and Z bosons through the weak interaction. This sheds new light on the question: how “strange” the proton is at small x?
The W production is detected through its decay into a charged lepton (electron or muon) and a neutrino, while the Z boson produces an electron-positron (or muon-antimuon) pair. The experimental detection of electrons and muons poses different challenges and thus the simultaneous measurement in both channels provides an important cross-check of the results, thus improving the final precision achieved. The integrated cross sections for Z boson and W boson production are measured with a precision of 0.3% and 0.6%, respectively, and with an additional common normalisation uncertainty from the luminosity determination of 1.8%. Differential cross sections are also measured in a variety of kinematic regions and about half of the measurement points have a precision of 1% or better.
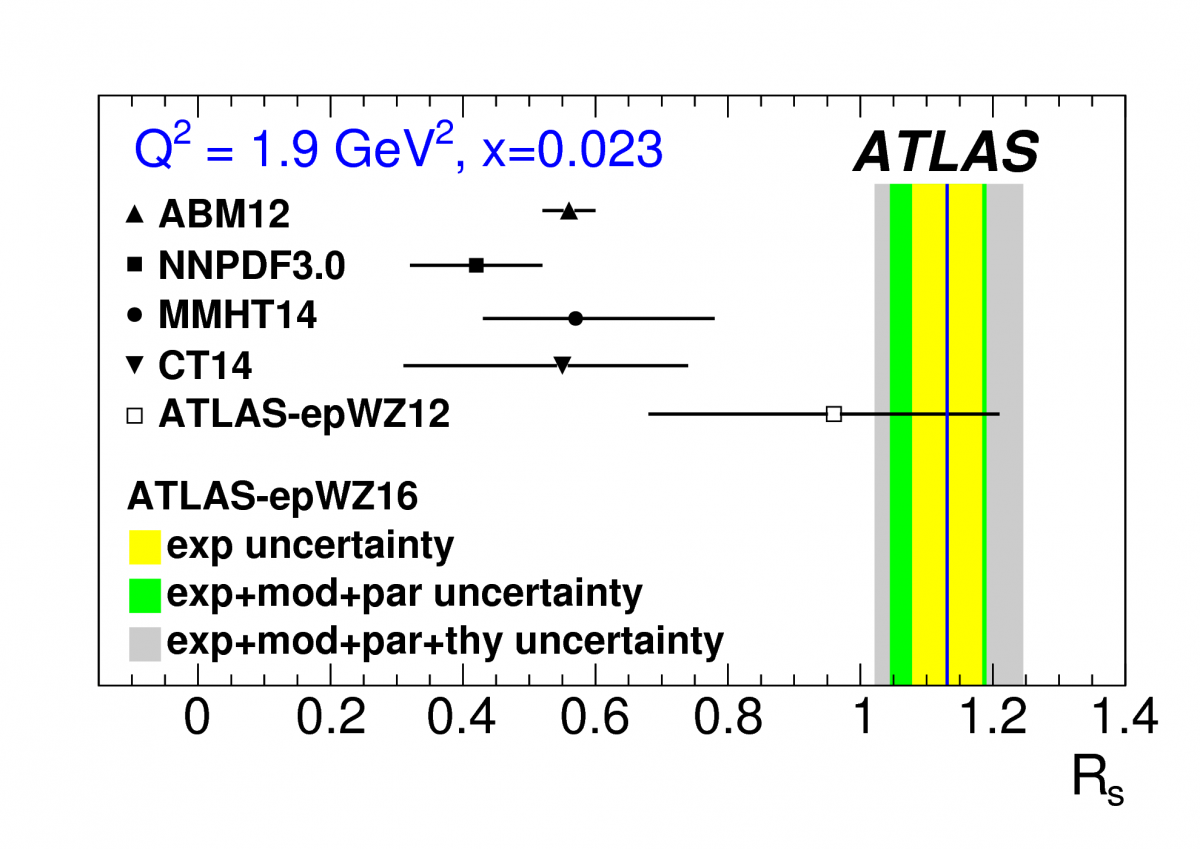
The measurements are then compared to state-of-the-art QCD expectations using different PDF sets. Because the production of W and Z bosons through the weak interaction has a different dependence on the specific quark flavours compared to the electromagnetic interaction seen in electron-positron scattering at HERA, analysing both data sets gives new access to the strange quark content of the proton.
As is shown in Figure 1, the measured production rate of W bosons is very similar for all PDF sets, in good agreement with the data. In contrast, the rate for Z boson production is underestimated significantly for most PDF sets. A dedicated analysis enables this deficit to be attributed to a too small strange quark contribution in most PDF sets. The new PDF set, which this paper presents, requires the strange quark sea to be of a similar size as the up and down quark sea. This is summarised by the quantity RS, which is the ratio of the strange quark sea to the up and down quark sea, and which is found to be close to one, as shown in Figure 2. This result is a striking confirmation of the hypothesis of a light-flavour symmetry of proton structure at low x. This result will generate many further studies, because hitherto there had been indications from low energy neutrino-scattering data that favoured a suppressed strange-quark contribution with respect to the up and down quark parts, leading to an RS close to 0.5.
The analysis also shows that the potential to which precise W and Z cross sections can provide useful constraints on PDFs is not limited by the now very high experimental precision, but rather by the uncertainty of the currently available theory calculations. The salient results of this paper are thus of fundamental importance for forthcoming high-precision measurements, such as the mass of the W boson, and also represent a strong incentive for further improving the theory of Drell-Yan scattering in proton-proton collisions.